a cubic millimeter is very small. It's hard to see – just a tiny speck. But if you look closely, you can discover a whole world inside. A team of neuroscientists and engineers, assisted by machine learning tools, have mapped a cubic millimeter of the human brain at nanoscale resolution, identifying every neuron, synapse, blood vessel, and supporting cell within the tissue and building a 3D model. Even though it represents just a tiny portion of the total brain volume, it is the most detailed map of a piece of human brain matter ever created. This could lead to a surge of scientific discoveries about neurological disorders, brain structure, and the origins of our behavior.
In one way, our data set is very small Jeff Licthman, co-senior study researcher and a neuroscientist and professor of molecular and cellular biology at Harvard University, tells PopSci. “But it doesn’t feel small because when you get in it, you see it’s like a gigantic forest. It’s a very tiny forest, but it’s a very, very, very complicated forest,” he adds.
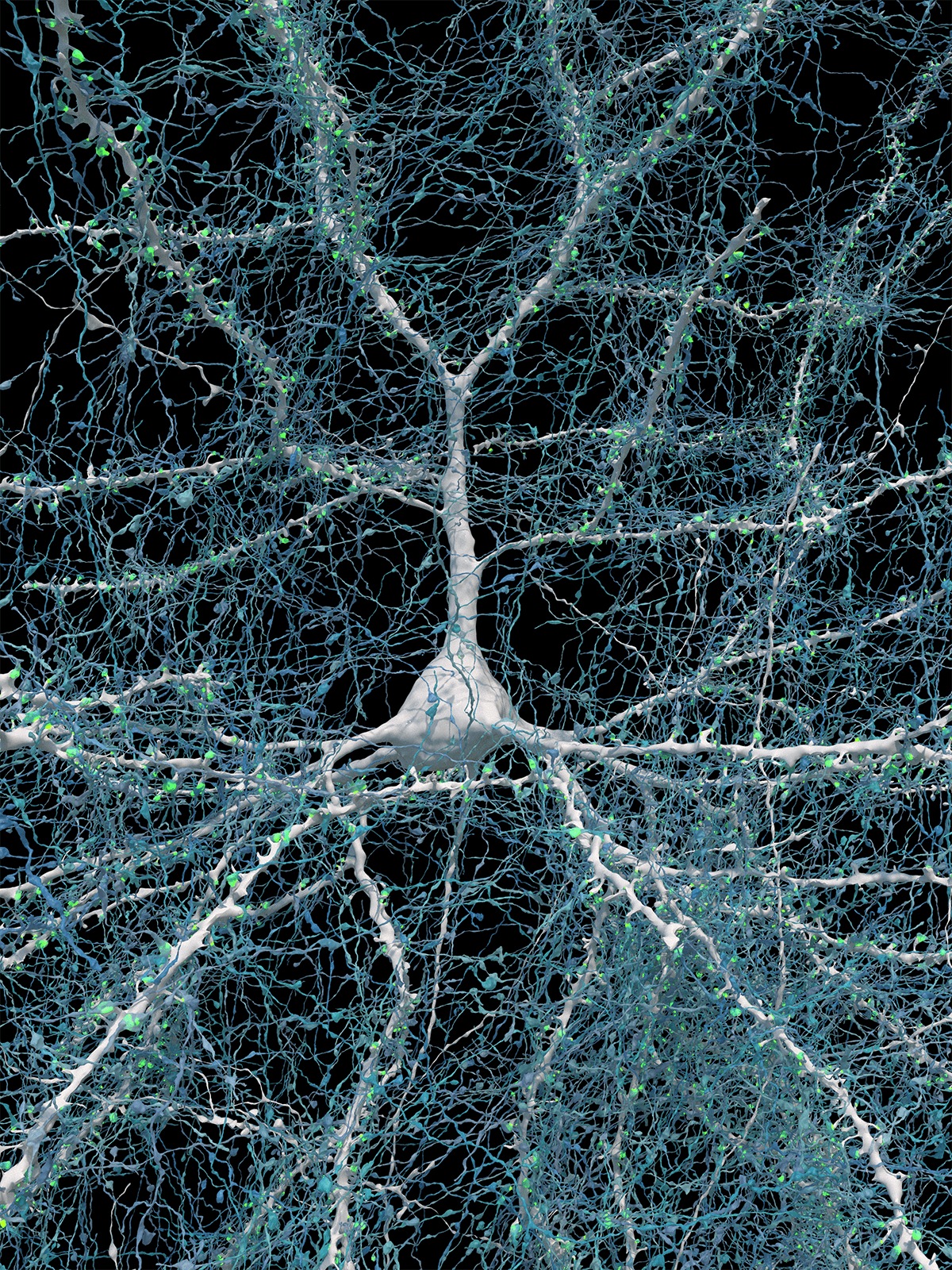
All that complexity is on display in a study documenting the construction of this bit of comprehensive brain map or “connectome,” published May 9 in the journal Science. The first connectome was of a nematode brain, completed in 1986. Since then, neuroscientists have continued to plot out increasingly large and complicated brains–including those of fruit flies, maggots, a tadpole, and an earthworm. Yet human brains pose a unique mapping challenge in their intricacy and inaccessibility. The new, partial human connectome is available online for anyone to explore.
“Not only is this an impressive technological feat, this is a tool and a resource that is really aimed at sharing with the world and getting all of this scientific information out there,” Tim Mosca, a neuroscientist at Thomas Jefferson University who was uninvolved in the new work, tells PopSci. “This group has done an amazing job designing all of the new tools and the pipelines to make this available to anyone who wants to look at it, wants to think about it, wants to use this in their research.
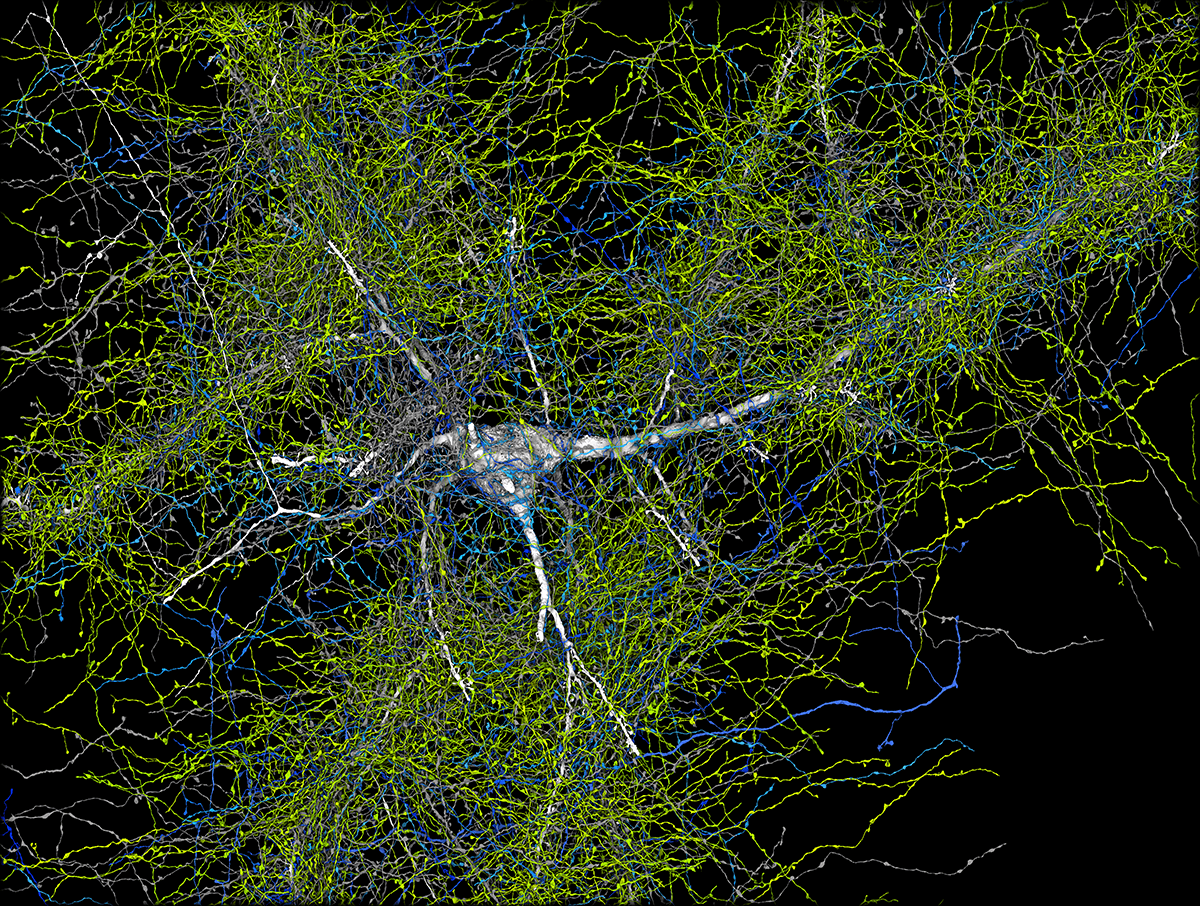
Serving up brain pizza
The study sample was collected over a decade ago from an anonymous patient undergoing epilepsy surgery. The surgeon removed a small piece of the temporal lobe to access and treat an underlying lesion, quickly preserved the tissue, and later shared it with scientists. Though the total volume of the fragment is about 1 cubic millimeter, it is not cube shaped. Instead, it's like a thick piece of pizza–but not that thick. This blunt, triangular chunk, longer than it is wide, enabled the researchers to capture a bit of all six layers of the 3mm thick cerebral cortex.
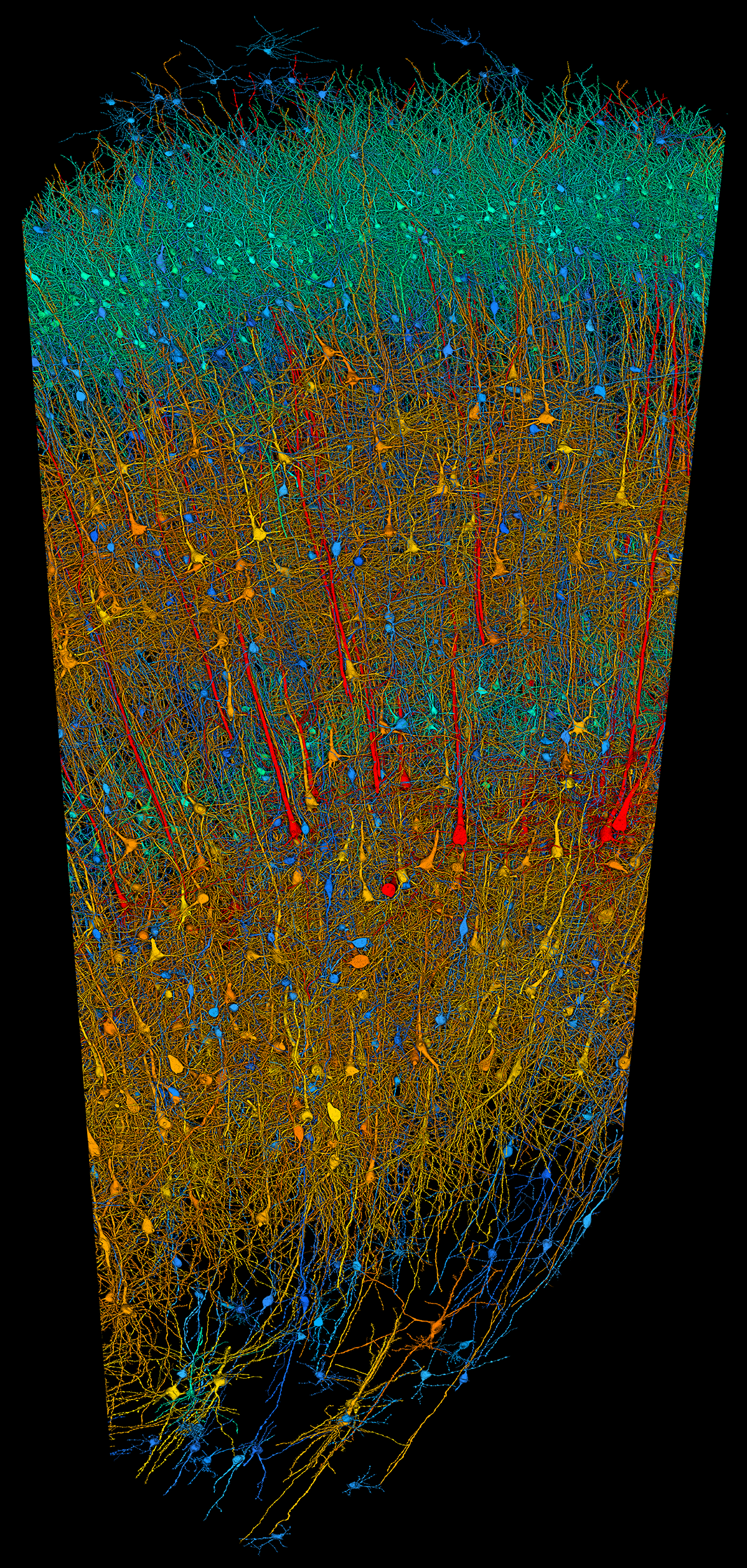
The first step to mapping the brain was to divide it into 5,019 individual cross sections, each 30 nanometers thick, and then mount them on tape using a specially designed machine with a diamond knife for cutting. slice it From there, the researchers spent a whole year carefully imaging each slice using an electron microscope. After that, they digitally aligned and stitched together the slices and used various machine learning tools to complete the 3-D shape and label and color each part.
The density of neurons in the segment is 16,000 neurons per cubic millimeter, which is around one-third lower than a previous estimate and 10 times less dense than the corresponding section of a mouse's brain. Glial cells, which hold brain tissue together, outnumber neurons in the fragment by a two to one ratio.
Neural explorers
While the brain fragment's physical size is small, the level of detail in the mapping effort results in massive amounts of data. The reconstructed segment is 1.4 petabytes in digital size, which is equivalent to about 1,400 terabytes or the storage capacity of 2,800 average laptops. There is a lot to potentially discover within that data, such as individual neural circuits, previously unseen cellular ratios and shapes, the composition of each cortical layer, and more.
Lichtman says, "It's like being an explorer that lands on a new island. You keep looking around and you're just going to keep finding new things."
Lichtman and his co-researchers have already made some interesting observations. Among the approximately 150 million synapses they mapped, they discovered a rare type of very strong connection. In the vast majority (96.5%) of cases, axons—the outgoing transmission line of neurons—formed one connection with a target cell. Some (about 3%) made 2 connections. But less than .01% formed more than four synapses, including some axons and target cells that were connected at over 50 points.
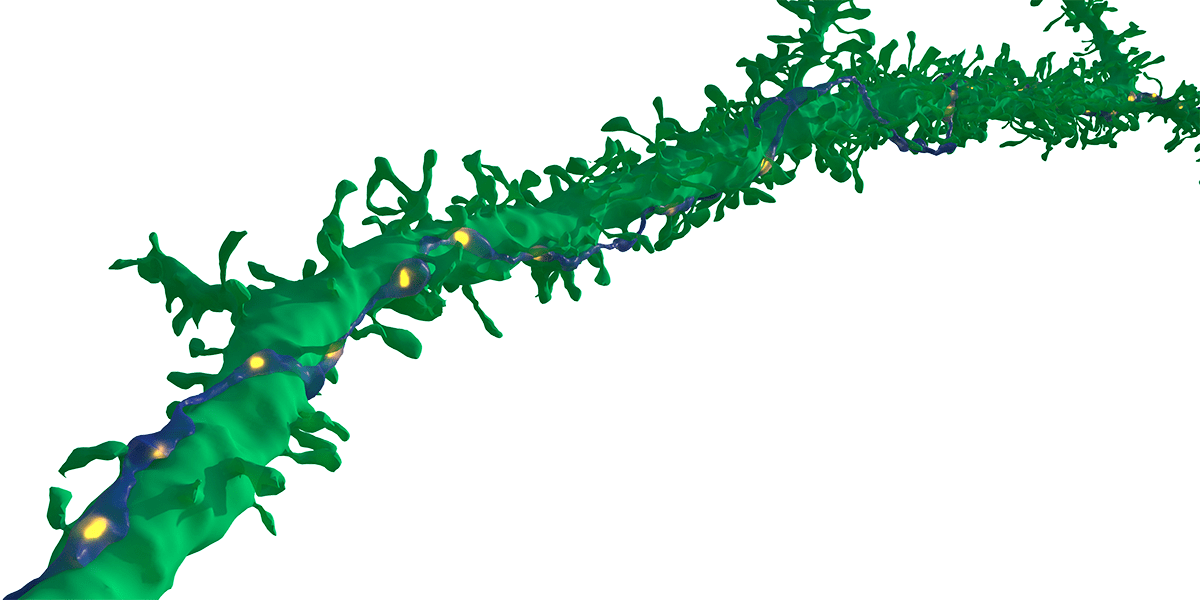
Mosca says, "We've always had a theory that there would be super connections, if you will, among certain cells. But it's something we've never had the resolution to prove… Now we know that it exists and we can investigate what it does." Lichtman's current hypothesis is that these extra-strong connections are the type of ultra-fast pathways that enable automatic use of the brain for well-established, learned actions.
Scientists made a new observation: many dendrites, which receive inputs, appear to point in symmetric directions, which is unexpected and mysterious.
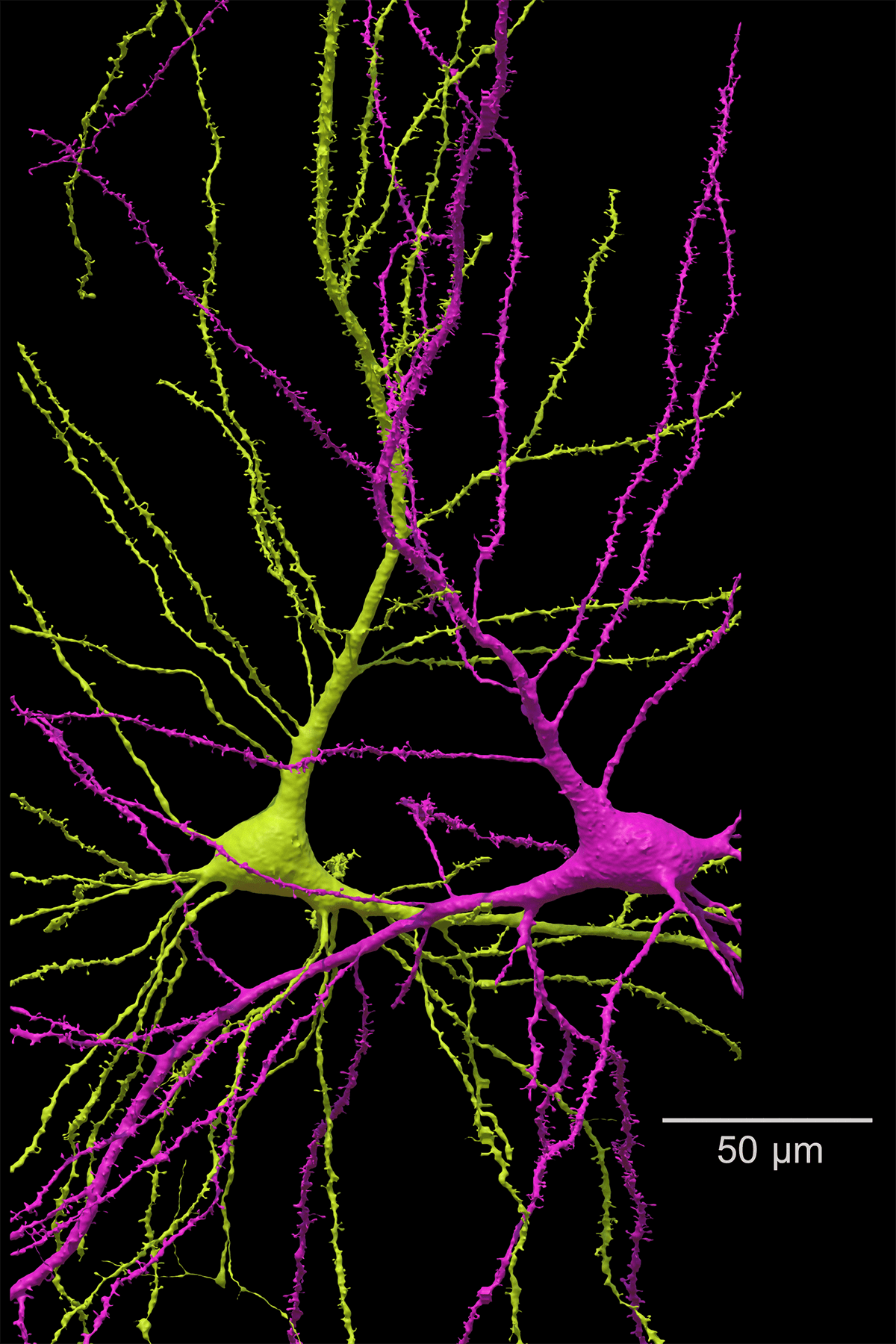
The researchers also found a new unexplained structure called an “axon whorl,” where long axon cables seem to tangle around themselves. Some axons had multiple knots, which was unexpected. Viren Jain, co-senior study author and a senior staff scientist at Google where he leads the company’s Connectomics research team. Again, the function and cause of these whorls is unknown. “We were not expecting to find such a structure. It’s very peculiar… like a big jumble of wiring that sort of contravenes the purpose of a wire to begin with, which is to go places and contact other things.”
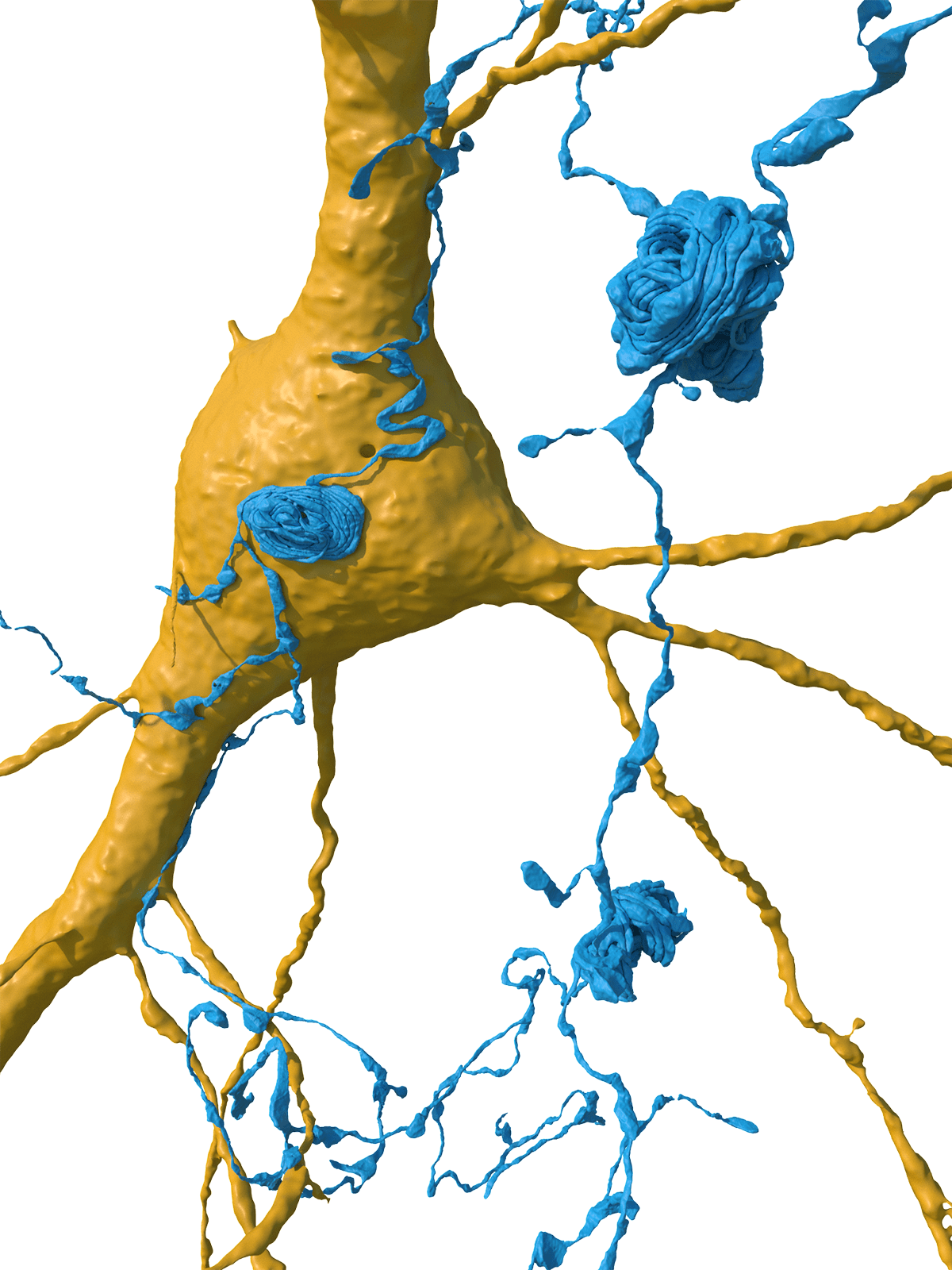
These three findings are likely just the beginning. The dataset is so large that it requires a collective effort to explore it thoroughly. 200 papers has cited the brain reconstruction since it was first released as a pre-print, Jain points out.
The partial connectome could eventually aid in understanding and treating brain diseases. “The ability to measure neural wiring of human brains in such detail opens up exciting opportunities for advancing human health,” says Andrew Leifer, a physicist and neuroscientist at Princeton University who wasn’t involved in the project. “One could imagine comparing different brains to understand how brain wiring changes when a healthy brain suffers from a disease or falls into dysfunction,” he adds.
Looking ahead
But while there is much to discover, there are also limitations. The automated machine learning methods used carry a margin of error that requires human oversight. Editing will be an ongoing project, and is a community science effort that anyone can apply to participate in.
The sample is also only one small piece of one person’s brain. There is much that can’t yet be inferred about human brains generally or other brain regions beyond the temporal lobe based on this single fragment without more samples and maps for comparison, notes Lichtman.
And, perhaps most importantly, the brain section came from someone having surgery for epilepsy. It might not show a “normal” brain and we can't be certain unless we have more pieces to examine, according to Jain and Lichtman. But we are planning many follow-ups to this,” Jain adds.
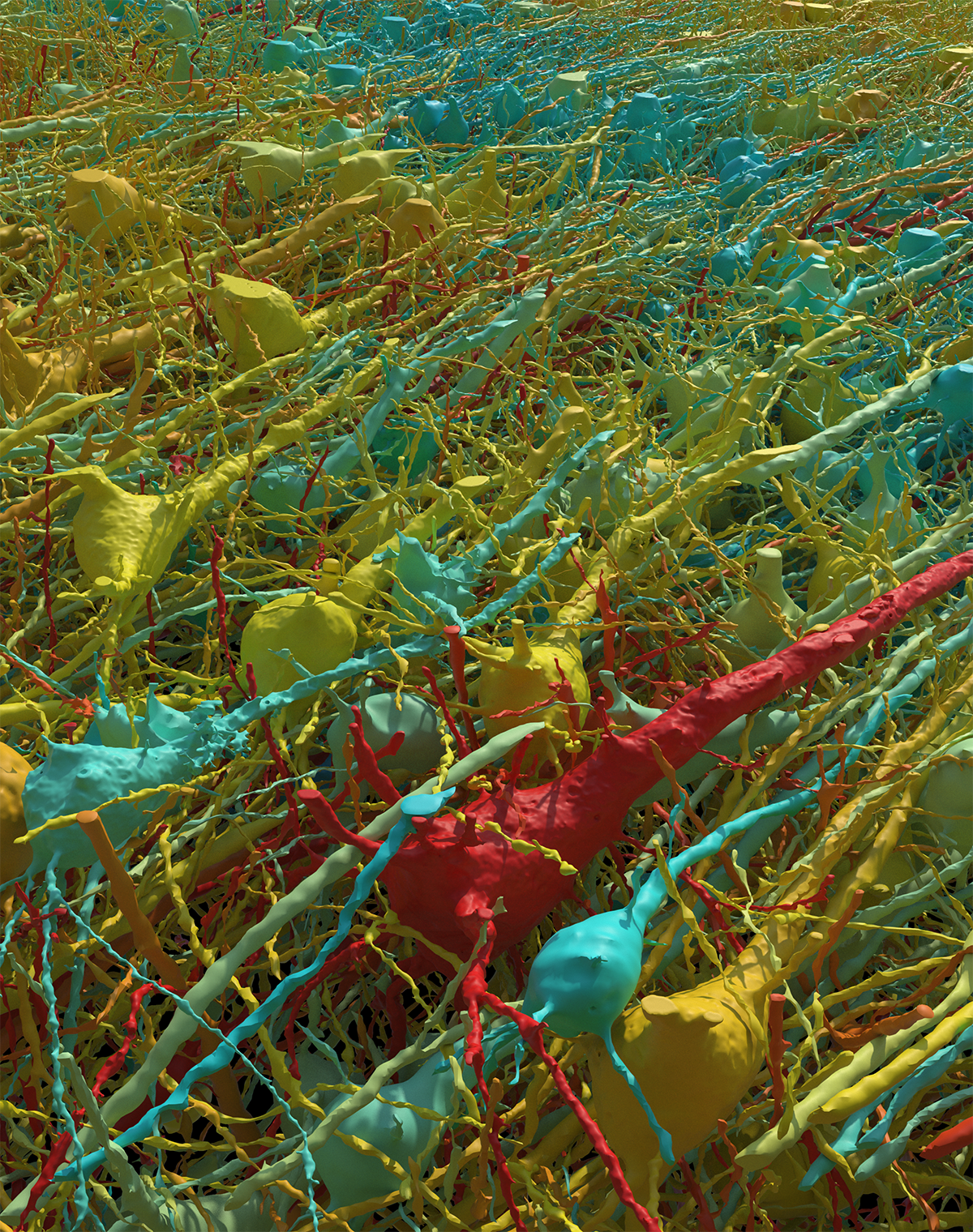
The team aims to create several incomplete connectomes representing more human brain samples. They are also working on zebrafish connectome, and are planning to investigate increasingly large parts of the mouse brain. Mammal brains have many similarities, so a comprehensive mouse connectome could provide new insights into our own brain and the development of brains across animals, Lichtman says.
Currently, with the technologies available (and the ethical concerns), a full connectome of the human brain is “a bridge too far,” says Lichtman. “Literally, we’re a million times away from that,” says Jain. But through this study, the scientists have taken an early (if tiny) step in that direction, and even the smallest peek can lead to a whole universe of knowledge.
“I would like people to consider this in the same way they think about the Hubble or James Webb telescope,” says Lichtman. “We’re looking into an unknown area, and one that is much more relevant to us than distant outer space. It’s this inner space that each of us have on our shoulders that we use, but know almost nothing about.